|
Haliotis varia (Linnaeus, 1758)
Variable abalone
|
|
Erin Jane Watson 2017
|
|
|
Summary | |
Haliotis varia ‘Common ear shell or the Variable abalone’ is one of the 56 species within the genus Haliotis, meaning ‘sea ear’ and characterised by the five to six open respiratory pores in the flattened ear shape shell (Brusca et al, 2016). It is smaller in size than most, reaching a maximum shell length of approximately 80mm.
This species is typically located in reefs and rocky shores along the coastal temperate and tropical shallow waters of the Indo-Pacific, including the Great Barrier Reef, with smaller populations located in some colder waters. The large muscular foot and dorsoventrally flattened shell allow the abalone to inhabit harsh water locations, where most of its competitors struggle to survive.
Reproduction occurs via broadcast spawning, and larvae transition from a free swimming planktonic phase into a juvenile occurs via settlement and metamorphosis (Balkhair, 2016). Once the transition has occurred, the abalone lives a sedentary lifestyle, using chemical signals to detect food, avoid predators and aggregate to reproduce (Roberts, 2013). Like most abalone, it is a nocturnal feeder, with a diet of red and green algae, using the radula to rasp away at the diatoms (Hickman, 1980).
Classification:
Kingdom: Animalia
Phylum: Mollusca
Class: Gastropoda
Clade: Vetigastropoda
Superfamily: Haliotoidea
Family: Haliotoidea
Genus: Haliotis
Species: Haliotis varia
|
|
|
Physical Description | |
Figure 3: A simple sketch of H. varia. Modified from Crofts, 1929.
Haliotis varia are asymmetrical, occupying a dorsoventrally flattened spiralled shell, with a wide ventral opening for the body where, typically only the brown thick, muscular foot is exposed and can be withdrawn into the shell when necessary.
Several respiratory pores on the outer edge of the shell are characteristic of all abalone, used for respiration, reproduction and waste removal (Crofts, 1929). The dorsal side of the shell and the epiodium (frill of tissue above the foot) is often banded black and white, however, the colours could vary in regards to the individual’s diet (Padilla, 1992). The shell is also provides a habitat which is favoured by encrusting algae species or small polycheates, which would mask the original colour of the shell, also aiding in camouflage. The colour of the shell, unless cleaned, is usually not suitable for species differentiation. If the flesh is removed, the underside of the shell is white nacre (mother of pearl) in design.
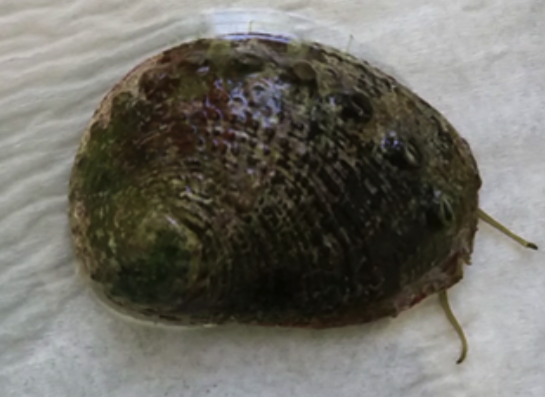
Figure 4: A H. varia specimen from the University of Queensland. An example of organisms on the outer shell masking the true colouration. Note the thin cephalic tentacles with dark coloured chemoreceptors located at the tip and the six open respiratory holes on the top of the shell. Scale 1:1
Like other species of abalone, H. varia possesses three specific sensory tentacles. Both the long pair of cephalic tentacles and the short pair of eye stalks are located anterior to the body. The epipodial tentacles are much smaller in size and are evenly distributed on the fringe of the mantle tissue. Initially, when crawling over substrate only the cephalic and epipodial tentacles are elongated and only once considered in a safe environment, the short eye stalks will extend to aid in navigation.
H. varia is differentiated from the other two tropical abalone found within the Great Barrier Reef (H. asinina and H. ovina) by the overall smaller size then the others and less elevated and less distinct ledge of the tremata. The shell shape is less elongated then H. asinina, and less round the H. ovina.
This species of abalone grows to a maximum size of 80mm, one of the smaller species in tropical Australia.
|
|
|
Ecology |
Predators | |
Haliotis varia reproduces by broadcast spawning, with no parental care or protection from the parents (Balkhair, 2016). The most vulnerable and predated phase within the abalone is during the early stages, with a mortality rate of approximately 85%, with predators and environmental conditions equally contributing to the mortality.
Predators:
Abalone larvae, like other marine larvae, are small and plentiful and are a favoured food resource by fish and larger filter feeders. If larvae have successfully undergone settlement and metamorphosis, to develop into an adult, the most threatening predators are fish, lobster, crabs, octopus and starfish (Aguirre, 2013, Balkhair, 2016, Roberts, 2013). Humans, generally, are the most threatening predator of all abalone species as the meat is an expensive delicacy, although due to the small size, H. varia are not generally targeted for fishing.
Predatory defence:
If an adult abalone detects a predators presence, it clamps the shell securely on the substrate using the strong muscular foot, so virtually no soft tissue or sensory organs or the abalone are exposed. Juvenile abalone’s muscles are not as developed, and they opt to hide within crevices or underneath rocks to avoid predator contact (Crofts, 1929).
Abalone may also choose to use the muscular foot and crawl attempt to escape the predator.
|
An example of predatory escape behaviour of abalone. Note that this particular species is not located within the Great Barrier Reef, however the behaviour from both the predator and abalone is similar to species which are distributed in the GBR.
Source: Shape of Life
|
Predatory impacts:
Predators often control the distribution and abundance of the prey population, especially in a prey species with limited predator defence strategies, such as the abalone (Aguirre, 2013). In addition, the environmental requirements for larval settlement also constricts the appropriate habitat in which an abalone can successfully develop, therefore controlling the demographics of the population (Roberts, 2013).
|
|
|
Habitat | |
Haliotis varia are found in tropical and temperate reef ecosystems, usually underneath boulders and in rock crevices (Padilla, 1992). They feed on algae and other diatoms which require nutrient rich, shallow waters and substantial sunlight, restricting H. varia to populate these waters, and are rarely found at depths greater than 40m (Degnan et al, 2006). If the water quality is poor and nutrient deficient, the abalone becomes stressed, growth rate decreases or abalone relocate (Burke et al, 2003).
Throughout the reef ecosystem, they are found in the inner reef flat, shallower waters, associated with sea grass, usually at depths of 5-10m and in some areas of the reef crest, as their flat shells and strong muscular foot allows them to attach to rocks. This allows them to populate turbid water and possibly outcompeting other grazing species that do not have the ability to survive in these harsher environments.
Figure 5: An example of the rock crevices which H. varia inhabit throughout the Great Barrier Reef. Note the organisms inhabiting the outer shell of the abalone, which also aid in camouflage from predators.
Figure 6: An intertidal reef flat on the Great Barrier Reef, a common habitat of H. varia.
Source: sanctuarysimon.org
|
|
|
Ecological Importance | |
Marine grazers, such as abalone, are an important aspect in the structure of the ocean community, by partially controlling the benthic ecosystem and supplying habitat in the form of their shells.
They have a significant influence on the food web because of their position within the community. The effects of herbivore grazing depends on the abundance of H. varia and their competitors. Grazing has direct effects on the algae and has indirect effects on competing herbivorous species and the predatory species of H. varia (Shepherd, 1986).
For example, consumption of grazers by predators can influence the abundance of algae and other benthic plants. This occurs when predators stop them from grazing by confining the abalone into hiding or by overconsumption to drastically deplete the population. This in turn would allow competitor grazers to increase in numbers and change the structure of the community.
In addition, if populations of H. varia were reduced or depleted within an ecosystem, it would have severe knock-on effects. For example, the interspecific and infraspecific completion would significantly increase, both in species higher up on the trophic level and within competing species. Impacting the natural balance in the environment.
|
|
|
|
Life History and Behaviour |
Reproduction and Life Cycle | |
Reproduction:
Like all abalone, H. varia are gonochoristic, broadcast spawners, releasing their eggs and sperm into the seawater allowing fertilisation to occur (Burke et al, 2003). When adults are sexually mature, they aggregate just before the spawning occurs, a behaviour that is only observed during breeding season, and rarely, if ever, in sexually immature juveniles (Shepard, 1986). This aggregation behaviour is also more pronounced as individuals age and become increasingly sexually mature, to maximise the contact between eggs and sperm and increase fecundity levels (Najumdeen, 2004). In addition, to aid fertilisation, males and females synchronously release eggs and sperm into the surrounding seawater, assumed to be a technique developed by evolution.
H. varia reach maturity at a relatively young age and small size, with the amount of eggs and fecundity increasing as they increase in size (Shepard, 1986, Najumdeen, 2004). Adult females release on average between 70 000 - 80 000 eggs per spawn.
Life cycle:
Once the sperm and eggs have successfully come into contact and fertilisation has occurred, the zygote develops into a free swimming trochophore larvae and gradually develops into a lecithotropic veliger larvae, supplied with a substantial amount of yolk to last them until they develop their own feeding structures (Balkahair, 2016). This nutritional resource allows them to disperse long distances, this technique avoids the risk of competition for resources between parent and offspring, and potentially also avoids inbreeding.
The transition from trochophore to lecithotropic larvae is characterised by the formation of the velum. The free-swimming larvae then undergoes settlement and metamorphosis, to transition into a crawling benthic juvenile - a process which is initiated by specific environmental chemical cues (Roberts, 2013). Metamorphosis is defined by the shedding of the velum, developing a muscular foot, ctenidia and increased growth rate of the shell (Balkahair, 2016).
Figure 7: The life cycle of Haliotis. Modified from Brusca, 2016
It is during this life stage when the abalone are most vulnerable, with mortality rate approximately 85-100% during the first two months (Balkahair, 2016). This is largely due to filter feeding predators and fish which indulge in abundant prey. In addition, settlement and metamorphosis are only possible if the abalone larvae is able to successfully detect the right environmental cues which initiate the transition (Roberts, 2013). However, if the larvae fail to recognise the correct cues, they either deplete their yolk source without settling and die, or when they deplete their yolk source they metamorphose without settling and mostly likely also perish (Roberts, 2013).
The development of the first respiratory pore within the shell is evidence that the metamorphosed larvae has transitioned into the juvenile stage, and when the abalone reaches sexually maturity, it indicates that it has reached adulthood (Balkahair, 2016).
|
|
|
Feeding | |
H. varia graze on attached algae or raise their shell and foot in an attempt to trap unattached algae (Crofts, 1929). At night, they abandon their sedentary lifestyle and move out of rock crevices and out from underneath coral shelters to feed.
Like all abalone, H. varia utilise the unique radula apparatus, a ribbon of chitinous teeth, which rasps away at algal diatoms and transports them into the buccal cavity. The abalone’s feeding habits changes throughout the abalone growth and development as a result of the development of the radula apparatus and ontogenetic changes in the digestive enzymes (Allen et al, 2006).
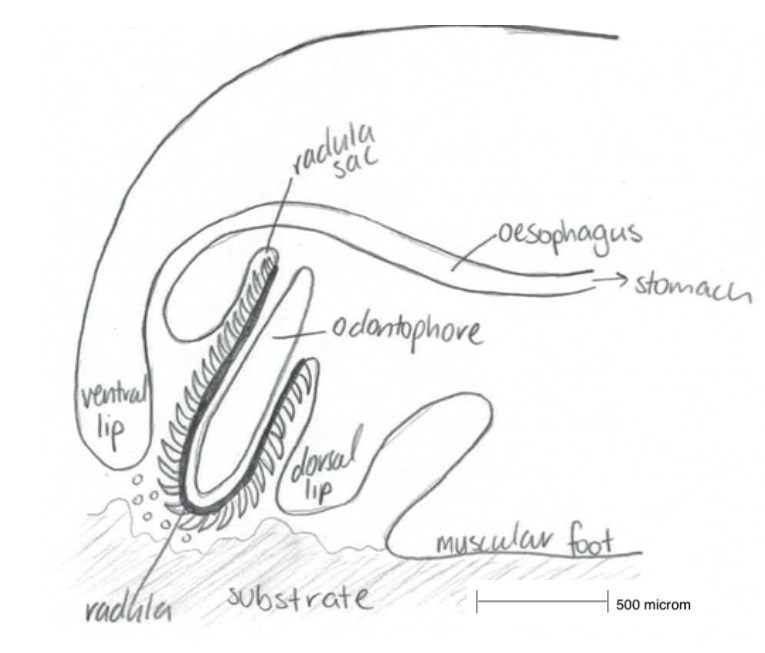
Figure 8: A simplified sketch of the action Haliotis radula. Modified from Crofts, 1929.
Larvae are non-feeding, and instead are supplied with a nutritional yolk within the egg, which supports them until they settle and begin metamorphosis. The process of metamorphosis transitions the free swimming, non-feeding larvae into benthic, feeding juveniles which must now feed on small particles, and not yet on algae, as the gut lacks a grinding mechanism at this stage (Hickman, 1980, Kawamura et al, 2001). Several days after settlement, the post-larvae abalone have approximately between 25-30 transverse rows of radula teeth (Johnston, 2005, Kawamura et al, 2001).
Another feeding change occurs when the shell reaches 0.6-0.8mm in length, whereby the abalone can now digest larger diatoms which increases the growth rate and efficiency of the abalone (Johnston, 2005). The transition from a diet biofilm to macro algae is the final feeding change, which occurs when the radula has completely developed and the abalone digestive system is capable of absorbing larger particles (Takami, 2003).
|
Haliotis varia feeding behaviour. Individual from the University of Queensland
Source: Author |
|
|
|
Radula analysis | |
Both the herbivorous and carnivorous molluscan diet and morphology requires the animal to physically place their mouth over their food in order to collect it (Strain, 2013).
The radula is a feeding apparatus, unique to molluscs (with the exception of bivalves), described as a ribbon composed of chitinous teeth which protrudes from the buccal cavity onto the substrate (Cofts, 1929). It is essential for the molluscan feeding behaviour in the process of rasping algal and diatoms from hard substrates and transporting them into the buccal cavity in the mouth of molluscs (Takimi, 2003).
The complex radula ribbon is associated with the odontophore, a muscular structure which originates from the base of the pharynx in the buccal cavity (Johnston, 2005). Protractor and retractor muscles within the cartilages odontophore move the radula back and forth (i.e. in and out of the buccal cavity) and onto the substrate. As the radula is pushed outwards, it is pulled over the bending odontophore and is pressed up against the substrate. The small chitinous teeth rasp and sweep the small particles into the radula midline and draw them into the buccal cavity (Hickman, 1980).
The radula originates in the radula sac, where the radula teeth and membrane are produced by odontoblast cells, to replace the teeth that are broken off after wear and erosion (Kawamura et al, 2001), throughout the molluscan lifetime. To restore the lost or broken teeth, the anterior of the radula sloughs off and creates space for new, sharp teeth to take their place. New teeth are shaped and mineralised by epithelial cells anterior to the odontoblasts (Hickman, 1980).
The radula arrangement and morphology, although conceptually similar in each molluscan group, varies significantly to benefit the different diets and feeding habits of the individuals (Degnan et al, 2006).
Abalone are dorsoventrally flattened, single shelled, herbivorous sea snails with several characteristic respiratory pores on the edge of their shell. They feed by either rasping off algae with their radular apparatus or by trapping unattached algae and drawing it into their buccal cavity (Cofts, 1929). The specific algae which abalone favour have a complex structure and requires equally complex radula structure to remove it from the substrate (Steneck, 1982). Abalone enjoy a diet of coraline algae, densely packed, thick-walled cells. Due to this, abalone require a particular radula structure to rasp away at the algae (Steneck, 1982).
Abalone radula are constructed in arhipidoglossate design, differing from other molluscan radula’s whereby the radula is protrusible with a central strip of large teeth (rachidian), with a long band of smaller, finer teeth on either side (Hickman, 1980). The lateral and central teeth rasp along the substrate, while the marginal teeth collapse to gather the food. Marginal teeth collapse is essentially the teeth folding over the larger lateral and central traps the food particles as the odontophore retracts into the buccal cavity (Cofts, 1929). During this folding of the radula, the teeth interlock to prevent any damage and misalignment of each individual tooth,
as teeth which are not interlocked when the odontophore protrudes are easily ripped from the cartilage and the radula damaged (Padilla, 1992).
Each individual radula tooth has three main compartments; i.e. base, shaft and cusp, described as follows (Hickman, 1980).
- Base: Attached to the underlying chitinous ribbon. The morphology is most modified relative to the mechanics and function of the radula. An enlarged base has the capability to perform multiple functions simultaneously.
- Shaft: The main purpose of the shaft is to withstand the compressional and tensional shear stress which each tooth is subjected to during feeding.
- Cusp: The only section of the tooth which contacts the food particles, hence, it is evolutionary diverse between all molluscs as the morphology is proportional to the type of food and substrate it is exposed too. The cusp is the termination of the tooth, it is triangular in shape and concludes in a sharp point.
The variation in tooth cusp, shaft and base size and length change with the different functions, lateral teeth are longer with narrow bases of attachment to rasp off the, whereas the marginal teeth are wider and shorter to sweep all the loose particles into the buccal cavity (Padilla, 1992).
Teeth which are highly pointed are more efficient at tearing and piercing algae, by means of concentrating the shear stress on the substrate compared to blunt teeth teeth, as the force is distributed over a wider area (Hickman, 1980). Hence, blunter teeth are more efficient at rasping loose material from the substrate. The teeth length also play a role in the function and efficiency, for example, shorter teeth are not as flexible and do not bend as easily as longer teeth of the same width (Cofts, 1929). Each anterior tooth will be used in feeding, until it is gradually worn down and breaks off to allow for a new, sharper tooth to replace it.
Although abalone generally have the same diet and feeding mechanisms, any variability can be caused by genetic polymorphisms or phenotypic plasticity (Degnan et al, 2006). Variation in the radula is an inexpensive and accessible to study and identify the difference in species and contrast in morphological functions (Lindberg, 2988).
Using literature, I aim to provide an example of how the radula can be assessed to identify the morphological differences between three of the 56 species of tropical abalone found in the Great Barrier Reef, in the genus Haliotis (Family: Haliotoidea), H. varia, H. ovina and H. asinina, with further investigation and dissection of H. varia.
Haliotis varia
This species was examined in the University of Queensland laboratory, whereby the feeding
behaviour was monitored and a dissection of the radula allowed for further examination into the feeding mechanism. Upon examination, it is concluded that the asymmetrical radula consists of a large central bicuspid rachidian tooth, surrounded on both sides by five lateral teeth with large numbers of marginal teeth tightly folded, the number of which in unknown, however from examining pictures, it is suggested that it has fewer teeth then the other two species. Lateral teeth decrease in size from the third to the fifth tooth.
The shell length of the individual dissected was 45mm and the length of the radula was 14mm, of which two thirds was folded.
Figure 9: The central rachidian flanked on both side by five lateral teeth, followed by numerous rows of marginal teeth, viewed from a dissection microscope, labelled accordingly. The marginal teeth are minuscule in size, unable to differentiate the number of rows.
Source: Author
Haliotis ovina
Chitramvong et al (1998) also examined the radula of H. ovina, describing that the relatively short central rachidian tooth is very similar to H. asinina, being asymmetrical and flanked with 5 lateral teeth and a very large number of marginal teeth. The transverse rows however, are larger in number, with a range from 225 to 247. The shape of the first lateral tooth is broad and blunt, with the lateral denticles of the marginal teeth being long and narrow (Chitramvong et al, 1998).
Figure 10: Scanning electron micrographs of H. ovina radula. Note the bicuspid rachidian, flanked on both sides with five rows of lateral teeth, followed by rows of marginal teeth, too minuscule to identify.
Source: Homburg, 2010
Haliotis asinina
From past experiments and literature, it is concluded that the asymmetrical radula is comprised of a unicuspid, short, broad, blunt and relatively large rachidian tooth, with five lateral teeth, each side of the rachidian and multiple marginal teeth, folded tightly. Chitramvong et al (1998) described the radula morphology of H. asinina in depth, illustrating that the lateral and central teeth are unicuspid, whereas the marginal teeth are mulitcuspid. The number of transverse rows of teeth range from 119 to 139. The shape of the first lateral tooth is long and slender. All teeth are lacking basal denticles and the base of the central tooth is greatly curved, creating a vertical groove (Chitramvong et al, 1998).
Figure 11: Scanning electron micrographs of H. asinina radula. Note the five rows of lateral teeth on each side of the central unicuspid rachidian. The number of marginal teeth rows are identifiable, due to the folding and small size.
Source: Homburg, 2010
|
Haliotis asinina feeding
|
All three of these tropical species are found in essentially the same locations and habitats around the Great Barrier Reef, and they eat generally the same diet. However, their radula differ in the number of marginal teeth, the shape and denticles in the lateral teeth and the size of the rachidian. Therefore from examining literature and conducting a dissection, it is plausible to determine that different species of abalone have evolved differences in their feeding apparatus, with the variation enabling more efficient feeding for each species.
|
|
|
Locomotion | |
Haliotis varia relies on the large muscular foot for movement, as it is composed of pedal retractor muscles, attached to the shell and the dorsal mantle (Shepherd, 1986). This muscle, along with several smaller muscles act together to raise and lower the muscular foot or to extend or shorten it, either longitudinally or latitudinally (Brusca et al, 2016). The foot moves in contraction waves, either in direct waves (back to front) or in retrograde waves (front to back). In direct wave movement, the contraction of the muscles begins at the posterior end of the foot, and the sequential sections of the foot follow. In retrograde waves, the foot contracts whereby the muscle is pulled forward in a front to back contraction wave pattern, movement begins in the anterior section of the foot (Brusca et al, 2016).
Haliotis varia demonstrate both feeding and non-feeding behaviours observed by the author in a laboratory environment.
Feeding behaviour:
- When feeding on a substrate, the mouth covers the food source with the radula moving in and out of the buccal cavity. The muscular foot expands and raises the shell, with the cephalic and epiodial tentacles extended and mobile.
Non-feeding behaviour:
- In the presence of a predator (or the author, in laboratory environments), H. varia holds the shell tightly to the substrate with the foot, cephalic and epiodial tentacles retracted.
- While on the search for food or other resources, H. varia has their shell raised, with all of the cephalic tentacles, including the eye stalks, and epiodial tentacles extended and actively motile, using chemosensory cues.
|
An example of the locomotion form of 'Retrograde Waves' in H.varia
|
|
|
|
|
Anatomy and Physiology |
Anatomy | |
Body wall:
The body wall of H. varia, like all gastropods is composed of three layers: cuticle, epidermis and muscles. The cuticle is primarily comprised of proteins and amino acids (Crofts, 1929). Specialised epidermal cells occur within the mantle, which produce the calcium carbonate material required to create the shell. The body wall incorporates there layers of smooth muscle fibres i.e inner longitudinal layer, middle diagonal layer and the outer circular layer (Brusca et al, 2016).
Epipodium:
The epipodium is a unique section of tissue which is located above and around the foot, coloured in white and black banding. This tissue is where the sensory epipodium tentacles stem from.
Mantle and Mantle Cavity:
The mantle is a thin organ which forms the dorsal body wall, growing during the abalone development and encompasses the body. This creates mantle folds which produce a space between the mantle and the body tissue, known as the mantle cavity (Brusca et al, 2016). Within the mantle cavity are the respiratory organs, as well as the location where the faecal and excretory waste is deposited to be collected by the exiting water current. After the developmental process of torsion, the mantle cavity lies above the head of the abalone (Shepherd, 1986).
Respiration:
As the water flows over the ctenidia (gills), oxygen is diffused from the water into the blood of the ctenidia. The blood travels to the heart, to be pumped around the soft tissue of the abalone (Cox, 1962). The tissues receive oxygenated blood, interchanges carbon dioxide into the blood. The carbon dioxide infused blood travels from the tissues back to the ctenidia, where the ctenidia release the carbon dioxide into the surrounding seawater (Brusca et al, 2016).
The ctenidia are located just above the head only on the left of the animal, where cilia beat to create a constant water current that enters through the respiratory pores in the shell and travels to the ctenidia (Cofts, 1929).
Figure 12: The respiration of Haliotis. The blue arrow indicates incoming and outgoing water. Green arrow indicates waste. Pink arrow indicates the reproductive gametes during breeding. Modified from Cox, 1962)
Digestion system:
H. varia diet consists of algae, which is rasped off the substrate via the radula apparatus through to the buccal cavity, down the oesophagus into the foregut of the stomach (Hickman, 1980, Takami, 2003). Within the stomach, the particles are sorted by size via the ciliated rigid sorting area, smaller particles are transported into the digestive glands upon the stomach wall to be digested extracellularly and larger particles are passed along the cilia and carried to the intestine (Allen et al, 2006). Abalone have a complete gut, therefore any food particles which are not absorbed are transported through the intestine and passed through the anus as faecal waste.
There are various glands that aid with the digestive process which are located within the anterior gut region, for example, salivary glands produces a lubricant which is secreted over the radula. Other glands produce digestive enzymes which aid in breaking up the particles (Johnston, 2005).
Waste system:
Faecal waste produced through the anus is deposited into the mantle cavity where the passing water current will transport it through the respiratory pores to exit the abalone.
H. varia possess two nephridia, in which the pericardial fluids (urine) pass through the nephrostome and into the nephridium, at this point selective reabsorption occurs in which any nutrients still remaining will be re-absorbed into the tissue and transported to the necessary location within the abalone (Takami, 2003). The remaining fluid is passed through the nephridiopore and is discharged into the mantle cavity, which, like the faecal waste is transported by the exiting water current.
Figure 13: The anatomy of Haliotis (Cox, 1962). Note the asymmetrical characteristic of the organs position and the lack of organs on the right side. Also note how the muscular foot takes up a third of the body, and how the organs wrap around it. All organs are located close to the ctenidia, allowing maximum dissolved oxygen.
Circulatory system:
The heart pumps blood through a simple, no musculature, circulatory system, delivering oxygen to the tissues and muscles of the abalone. The blood also transports the products of
the digestion process
from the absorption sites and delivers them throughout the body, as a section of the intestine is located within the ventricle (Russell, 1989, Brusca et al, 2016). The heart is located within a pericardial chamber on the left side of the visceral mass, with two atria (auricle)
symmetrically surrounding the single ventricle. The atria
receive oxygenated blood (which originates from the lungs) from smaller branchial vessels. From the atria, it travels into the muscular ventricle which, transports it through a large artery and through the rest of the body's tissues. Oxygen depleted blood travels from the tissues back to the heart and is then pumped through the lungs to replenish in oxygen (Russell, 1989).
Figure 14: The circulatory system of Haliotis. Note the intestine location within the ventricle to aid in absorption (Cox, 1962).
Nervous system:
There are numerous ganglia joined by nerve cords within the abalone, each controlling a specific region of the body, described as follows (Brusca et al, 2016, Cox, 1962);
- Cerebral ganglia: Controls both the peripheral and buccal ganglia. The peripheral nerves controls the nerves in the head tentacles and eyes. The buccal ganglia controls the buccal region, radula apparatus and oesophagus. The cerebral ganglion also controls the ctenidia, by the branchial ganglion connected to the cerebral ganglion via the tegumentary nerve.
- Anterior epipodium ganglia: Controls the nerves in the mouth and lips, skin and connective tissue, via the epipodial nerves and pedal commissure.
- Pleural nerve: Controls the nerves within the internal organs.
- Pedal nerves: Controls the ventrally paired nerves within the muscular foot.
In general, because the abalone is a primitive animal, the nervous system is simple when compared to other molluscs, as it is weakly concentrated with poorly formed ganglia. This nervous system is nevertheless sufficient for the sedentary lifestyle of the abalone (Brusca et al, 2016). Throughout the process of torsion, the posterior section of the nervous system is shaped into a 'figure 8.'
Figure 15: The nervous system of Haliotis. Note the 'figure 8' pattern of the plural-pedal ganglion mass, a consequence of torsion (Cox, 1962).
|
|
|
Sensory Organs | |
Water-borne chemical cues play a major role in most marine invertebrates, in all life stages, usually dictating the distribution and behaviour of the individuals (Kuanpradit et al, 2011). Abalone especially, as they are most active during the night and must rely on senses other than vision.
Larval form:
Larvae require detection of both chemical and environmental signals or cues which control where the larvae should settle and when metamorphosis begins (Baxter, 1992). Specific molecules, such as lysine and diamino acids increase inducers of metamorphosis in abalone, and control when that transition initiates (Roberts, 2013).
Adult form:
After metamorphosis, abalone have three types of tentacles, all of which help to receive chemical and environmental signals (Baxter, 1992).
- Cephalic tentacles (pair): located on the head of the abalone and are long in size when extended, bearing chemoreceptors.
- Optic (eye) appendages (pair): shorter and broader appendages, located on the outer border of the cephalic tentacles and bearing simple eyes.
- Peripheral epipodial tentacles: short, thin tentacles, in numerous numbers which surround the margin of the epipodium. These tentacles receive chemical signals from the broader environment.
Figure 16: The sensory organs and head of H. varia specimen in UQ, viewed under a compound microscope. Note the long and thin cephalic tentacles, the short and broad eye appendages and the short and thin numerous epipodial tentacles.
Figure 17: The eye of Haliotis. Note that there is no cornea, rather, the abalone utilises sea water for light refraction.
Source: Cox, 1962
The eyes are located at the end of the short eye appendage. They are cup-shaped with a spherical crystalline lens. There is no corneal lens, and therefore are submerged within seawater, which aids in the refraction of light (Cox, 1962).
Mating:
During the breeding season, abalone must aggregate to maximise fecundity between the spawned eggs and sperm. As abalone move, they produce a mucus from their epithelial tissue which encapsulates multiple chemical molecules (produced by the secretory cells), known as MAPs, which become synthesised and released into the seawater, acting as signals for other abalone to follow (Kuanpradit et al, 2011).
Once the congregation of abalone has occurred, eggs and sperm are typically released synchronously, however, tryptophan reserves in the females is then released, creating a chemical signal attracting the sperm cells to the eggs.
As abalone usually aggregate and spawn in areas of turbid water with strong currents, it is beneficial to use a molecule larger in size which will have a prolonged life span and allow large dispersal of the signal (Najumdeen, 2004).
Predators:
Specific predators of abalone release kairomones, which informs the abalone that a predator is present, allowing the abalone to respond by either hiding or increasing the suction onto the substrate (Mach, 2011). Typically, the closer the predator is, a greater number of kairomones will reach the abalone, thus, allowing it to respond accordingly.
Smaller sized molecules deteriorate quickly, and are thus used for communication in situations where rapid dispersal of the signal is required. Larger molecules require more time and effort to create, however are efficient in situations in which the chemical cue must remain functional without deterioration for longer periods of time (Kuanpradit et al, 2011). The cephalic tentacles receive the chemical signal and activate electrochemical receptors which then trigger the nerve cord, resulting in a response from the abalone, such as locomotion (Baxter, 1992).
|
|
|
Shell and Tremata | |
The shell of H. varia first develops during the larval stage, from extracellularly produced calcium carbonate which is laid down like 'brickwork,' with a protein matrix acting as the 'cement' (Brusca et al,
2016). This process of calcium carbonate brickwork is continued throughout the life span as adult abalone must constantly add to the existing shell. If the shell is removed, the abalone is able to continue surviving however, can not regenerate a new shell. The shell is originally produced by glands within the mantle that secrete the necessary materials and proteins required to create the hard, long-lasting substance (Hickman, 1980).
Figure 18: The shell of Haliotis. Notice the new tremata forming on the growing edge. Modified from Brusca, 2016.
H. varia, although has a very dorsoventrally flattened shell, spirals in whorls from the apex in a clockwise direction, with the tissue permanently attached via the muscle attachment (Brusca et al, 2016).
The protoconch is the original larval shell, where the initial whorls occur. As the abalone grows, the whorls increase in size, adding more and more material to the edge of the mantle. The largest whorl is the most recently produced, which includes the aperture for the head and tentacles (Cofts, 1929).
There are three layers to each of the shells; the outer peristracum, the middle prismatic and the inner nacre (Brusca et al, 2016).
- Peristracum layer: Controls the synthesis of the outer organic layer. This outer layer is rough and flat, allowing the abalone to inhabit areas such as reef crests, as they are able to withstand rough water, strong currents and waves.
- Prismatic layer: Consists of calcium carbonate, which directs the calcite crystallisation.
- Nacre layer: Consists of rhombohedral aggonitic crystals, is soft and smooth to protect the soft tissue.
Figure 19: The different shell layers of Haliotis.
Tremata (respiratory pores):
Haliotis varia typically have five or six pores in their shell, which they use for respiration, waste disposal and reproduction. As the abalone shell grows and spirals, new pores are formed and the older holes are filled in, to maintain the constant line of pores above the ctenidia (Brusca et al, 2016). The pores are located above the ctenidia, in which the cilia beat to create a constant current of water that enters through the pores to flow over the ctenidia. The water current also exits via the pores, which passes the anus and reproductive organs to removes any waste or disperse the reproductive gametes from the abalone (Crofts, 1929).
|
|
|
Torsion | |
Torsion is a characteristic unique to all gastropods during their developmental processes. It usually occurs during the late veliger stage, in which the visceral mass rotates 180 degrees counterclockwise (Brusca et al, 2016). The mantle cavity, visceral mass and anus are displaced from the posterior to an anterior position above the head. This distinctive process occurs in two steps with the first occurring during larval development, in which an asymmetrical foot retractor muscle develops and extends from the right, over the gut and anchors to the left side (Brusca et al,2016). In a quick contraction of this muscle, the shell and visceral mass are spun 90 degrees in a counterclockwise direction.
The second stage of torsion occurs at a slower pace, resulting from differential tissue growth. As a consequence of torsion, the gut is warped into a U-shape, with the anus and mouth at the anterior of the abalone (Brusca et al, 2016).
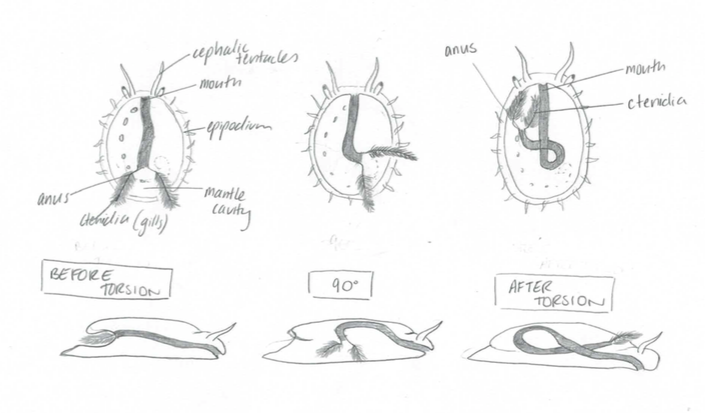
Figure 20: The developmental process of torsion. Note the initial position of the ctenidia and how it differentiates to the final position above the head. Modified from Brusca, 2016.
In addition, the longitudinal nerve cord develop into a figure eight design. The benefit of torsion is that the ctenidia are facing forwards on and receive fresh incoming water.
A disadvantage to torsion is that the anus is directly next to the ctenidia and all the waste and faecal matter enters the ctenidia if they aren’t creating a current strong enough.
|
|
|
|
Biogeographic Distribution | |
Haliotis varia are located in coastal temperate and tropical waters, with a large majority of the population situated around the Indo-Pacific region. The coastlines of Malaysia, Papua New Guinea and the northen coastlines of Australia. There are few locations further north and south in cooler waters, however warm water is favoured.
Figure 21: The known distributions (indicated in red) of H. varia.
Source: http://www.sealifebase.org/summary/Haliotis-varia.html
|
|
|
Evolution and Systematics | |
Gastropods are the largest and most morphological diverse taxa, with their behaviour, appendages and feeding apparatuses modified to benefit each group and their ecology. It has been suggested, that the first gastropods were not able to continually grown in length, which resulted in the body of the ancestral gastropod to develop the characteristic visceral hump. From fossil records, it is also assumed that the Pre-Cambrian ancestor of Gastropoda was asymmetrically single, coiled shelled. This feature of the asymmetry coiled shell restricted the right section of the mantle cavity and thereby, reduced or removed the structures on the right side. In addition, Gastropods evolved the process of torsion, pairs of cephalic tentacles and an operculum (a calcareous plate which covers the opening of the shell).
Within the Class Gastropoda, the Family Haliotididiae belong in one of the three Subclasses, Vetigastropoda, defined by morphological, Family specific characteristics. These include; a nacreous inner shell, rhipidoglossate radula for a herbivorous diet, one to two bipectinate ctenidia, two atria with a single ventricle and the loss of the operculum, differing from the other Gastropods (Geiger, 1999).
Current species of abalone differ in the shell colour and structure, epipodium and hypobranchial gland (Degnan et al, 2006), however, only the hard calcium carbon shell is fossilised.
In addition, the shell itself is variable throughout past and current species, differing in shell roundness, number of tremata and shell outline. In addition, the environment in which the abalone inhabits typically has an influence of the evolution of the morphological plasticity of the abalone’s shell, which could differ between and within species. It is difficult to determine the phylogeny of past and present
species of abalone without genetics.
Degnan et al. (2006) compared three widespread Indo-Pacific species (H. varia, H. ovinina and H. asinina) using molecular genetic techniques and determined that the positions of all three species within the phylogenetic tree are subject to change. It is also assumed that these three tropical species of abalone, diverged from the other temperate species. Results from the study suggested that H. varia is a sister taxa to H. dissona, a species from Tonga and that H. ovinina and H. asinina are more closely related as a sister taxon, with H. varia a sister taxon to that pair. Overall, the study gave a general phylogenetic position to each species, but the accurate position is subject to change.
Figure 22: The phylogenetic tree indicating evolutionary position of
H. varia, modified from Degnan et al. (2006). The red box indicates the general phylogenetic position of H. varia compared to the other species.
|
 |
Figure 1 |
|
|
|
Conservation and Threats | |
Overfishing:
Abalone meat is an expensive delicacy which supports a worldwide fishing trade to keep up with the demand for their meat. Haliotis varia being one of the smaller species is not a greatly impacted by fishing as consumers target the larger, more desirable species (Shepherd, 1993).
Fishing down the trophic food web can indirectly impact H. varia as it disrupts the ecosystem structure (Shepherd, 1993). For example, overfishing of some fish species may increase the abundance of the abalone’s primary competitor, the sea urchin (Strain, 2013). Thereby, increases competition for the same resources.
Figure 23: The result of overfishing of abalone in the 1920's, America
Source: https://briantissot.com/2014/09/07/the-hunt-for-monster-red-abalone/
Climate change:
Although climate change and global warming have currently multiple negative effects on the world’s oceans and especially the Great Barrier Reef, the most concerning for H. varia is ocean acidification (Harvey et al, 2014). Increasing levels of dissolved carbon dioxide within the ocean is causing the pH to decrease and the water to become more acidic. Species such as H. varia which form their shells using calcium carbonate will be the most vulnerable to ocean acidification as the calcification rate will decrease as well as the level of calcium carbonate saturation (Harvey et al, 2014).
As such result animals with calcium carbonate shell will struggle to continue growing and forming their shell*.
Larvae and juvenile stages of a marine invertebrates life are generally the most sensitive to environmental conditions (Balkhair, 2016, Crim, 2011). Ocean acidification can cause large impacts on the development, growth and survival in early life stages and consequently will have long term effects on both the individual and the population*.
Climate change is also resulting in species altering their distribution and range, due to the variation in environmental conditions (i.e. water temperature) (Harvey et al, 2014). As adult abalone are largely sedentary, it is difficult for them to adapt and relocate to their optimal conditions*. However, their primary competitor, the sea urchin, has demonstrated the ability to expand their range (Strain, 2013). This change in food source distribution and abundance and an increase in competition results in an increase of negative impacts.
The ICUN have currently not assessed the treats of H.varia, there are no known threats which are severely damaging population numbers, therefore no conservation methods are in place as of yet.
*This is assuming that the abalone can not evolve to the changing conditions
|
|
|
References | |
Aguirre, J., McNaught, D., 2013. Habitat complexity mediates predation of juvenile abalone by starfish. Marine Ecology Progress Series. 487, 101-111.
Allen, V., et al. 2006. The effects of tactile stimulants of feeding, growth, behaviour, and meat quality of cultured Blackfoot abalone, Haliotis iris. Aquaculture. 257(4), 294-308.
Balkhair, M., Almushikhi, A., Rivera, R., 2016. Embryogenesis and larval development of the omani abalone (Haliotis mariae wood, 1828). Journal of Shellfish research. 35(3), 625-631.
Baxter, G., Morse, D., 1992. Cilia from Abalone larvae contain a receptor-dependent G protien synthesis transduction system similar to that in mammals. Biological Bulletin. 183(1), 147-154.
Burke, C., et al. 2003. Environmental requirements of abalone. FRDC. 97(323).
Brusca, R., et al. 2016 Invertebrates, third ed. Sunderland, Massachusetts.
Chitramvong, Y., et al. 1998. Scanning electron microscope study of radulae in Haliotis asinina Linnaeus, 1758 and Haliotis ovina Gmelin, 1791 (Gastropoda : Haliotidae). Journal of Shellfish Research. 17,755-759.
Cox, K., 1962. California Abalones, Family Haliotidae. Fish Bulletin. 118, 20-26.
Crim, R., Sunday, J., Harley, C., 2011. Elevated seawater CO2 concentrations impair larval development and reduce larval survival in endangered northern abalone (Haliotis kamtshatkana). Journal of Experimental Marine Biology and Ecology. 400(2), 272-277.
Crofts, D., 1929. Haliotis. Liverpool Marine Biololgy. No 29.
Degnan, S., et al. 2006. Evolution in temperate and tropical seas: Disparate patterns in southern hemisphere abalone (Mollusc Vetigastropoda: Haliotidae). Molecular Phylogenetics and Evolution. 41(1), 249-256.
Geiger, D., Groves, L., 1999. Review of Fossil Abalone (Gastropoda: Vetigastropoda: Haliotidae) with comparison to recent species. Paleontological Society. 73(5), 872-885.
Harvey, P., et al. 2014. Evolution of Marine Organisms under Climate Change at Different Levels of Biological Organisation Water. 6, 3545-3574.
Hickman, C., 1980. Gastropod radulae and the assessment of form in evolutionary paleontology. Paleobiology. 6, 276-294.
Johnston, D., Moltschaniwskyj, N., Wells, J., 2005. Development of the radula and digestive system of juvenile blacklip abalone (Haliotis rubra) potential factors responsible for variable weaning success on artificial diets. Aquaculture. 250, 341-355.
Kawamura, T., et al. 2000. Radula development in abalone Haliotis discus hannai from larva to adult in relation to feeding transitions. Fish Science. 67, 596- 605.
Kuanpradit, C., et al. 2011. Characterisation of mucus-associated proteins from abalone (Haliotis) - candidates for chemical signalling. FEBS Journal. 279(3), 437-450.
Mach, M.E., Bourdeay, P., 2011. To flee or not to flee? Risk assessment by a marine snail in multiple cue environments. Journal of Experimental Marine Biology and Ecology. 409, 166-171.
Najmudeen, T., Victor, A., 2004. Reproductive biology of the tropical abalone Haliotis varia from Gulf of Mannar. Journal of Marine Biology. 46(2), 154-161.
Padilla, D., 1992. Functional consequences of feeding morphology and plasticity in an herbivorous gastropod, Lacuna. Journal of Animal Zoology. 32, 96A.
Roberts, R., Nicholson, C., 2013. Variable response from abalone larvae (Haliotis iris, H. virginea) to a range of settlement cues. Molluscan research. 18(2), 131-141.
Russell, C., & Evans, B., 1989. Cardiovascular anatomy and physiology of the black-lip abalone, Haliotis ruber. Comparative Physiology and Biochemistry. 252(2), 105-117.
Homburg, T., 2010. Radular morphology of Haliotis asinina, Haliotis ovina and Haliotis varia from the Great Barrier Reef, Australia: a comparative analysis. School of Biological Sciences, University of Queensland.
Shepherd, S., Brown, L., 1993. What is an abalone stock: Implications for the role of refugia in conservation. Canadian Journal of Fisheries and Aquatic Sciences. 50(9), 2001-2009.
Shepherd, S., 1986. Studies on the Southern Australian abalone (genus Haliotis). Journal of Marine Biology.90, 231-236.
Strain, E., Johnson, C., Thomson, R., 2013. Effects of a range expanding Sea Urchin on behaviour of commercially fished Abalone. PLoS.
Steneck, R., Watling, L., 1982. Feeding capabilities and limitations of herbivorous molluscs: A functional group approach. Journal of Marine Biololgy. 68, 299.
Takami, H., Kawamura, T., 2003. Dietary Changes in the Abalone, Haliotis discus hannai, and the relationship with the development of the digestive organ. JARQ. 38(2), 89-98.
|
|
|
|
|