Overview
Brief Summary
Common Names
Comprehensive Description
Biogeographical Distribution
Physical Description and Morpholgy
Features of a Planaxid Shell
Size
Shell Morphology
External Anatomy
Internal Anatomy
Apomorphy
Evolution & Systematics
Fossil History
Phylogenetics
Ecology
Local Distribution and Habitats
Micro-habitats and Associations
Life History & Behaviour
Larval Development
Reproductive Behaviour
Locomotion and Foraging Behaviour
Predator Avoidance and Escape Behaviour
Conservation
Trends and Threats
References & More Information
Bibliographies
Search the Web | Predator Avoidance and Escape Behaviour
Behaviour of Planaxis sulcatus in response to affluent of conspecifics
|
Background
In trying to study the behavioural predator-prey interaction of an organism, prey escape and avoidance response is an important aspect of this complex intra-specific interaction that’s needs to be well understood (Lam 2002). Potential prey will adapt avoidance responses well before it actually encounters the predator when chemical substances emitted by predators (i.e. kairomones) are detected at distances in order to escape being preyed upon by it (Jacobsen & Stabell 1999; Lam 2002). At close proximity, when the prey senses or is touched by a predator, the instantaneous reactions taken by the prey are defined as the escape responses (Lam 2002). Both types of behavioral responses can be chemically triggered and many aquatic animals rely heavily on their chemical sensory system to detect the presence of their predator (Jacobsen & Stabell 1999; McKillup & McKillup 1993).
Characteristic prey escape and avoidance response behaviours have been well documented in many species of marine invertebrates following detection of chemical cues emitted by predators or conspecifics, especially in marine gastropods species (Jacobsen & Stabell 1999; Lam 2002; McKillup & McKillup 1993). Gastropods have developed a suite of sophisticated chemoreception systems which allow them to detect and respond to chemical cues that indicates risk of being predated (Jacobsen & Stabell 1999; Lam 2002).Prey escape and avoidance response in marine gastropod species can ranged from increase movement rates, seeking shelter to avoid detection (i.e. self-burial) to movement upwards on vertical surfaces (negative geotaxis) in order to increase the chances of survival (Lam 2002; McKillup & McKillup 1993).
Many marine invertebrates, including gastropod species, were reported to be able to detect predators indirectly by responding to species-species signaling substances (i.e. pheromones) in the effluent from damaged conspecifics (Jacobsen & Stabell 1999; McKillup & McKillup 1993) These characteristic responses can vary vastly depending on the species involve (McKillup & McKillup 1993). For some, such as a number of hermit crab species, effluent from damaged conspecifics presents an opportunity to acquire a larger shell and hence they are attracted to it despite the risk of being predated (Rittschof et al. 1992). In many others, it has been suggested that the signaling substances in effluent is perceived as an alarm cue suggesting the presence of predators within the vicinity, triggering escape and avoidance responses to the stimulant (Jacobsen & Stabell 1999; McKillup & McKillup 1993).
This study aims to examine the behavior of Planaxis sulcatus in response to effluent of conspecifics collected from Heron Island on the Southern Great Barrier Reef, Australia under laboratory conditions. It is hypothesis that the effluent of conspecifics will trigger an escape response from P. sulcatus, similar to that observed in Fiji on the Heron Island population (McKillup & McKillup 1993).
|
Approach
This study was performed on the population of P. sulcatus inhabiting the beachrock on the Southside of Heron Island, Southern Great Barrier Reef, Queensland, Australia (23°26′31.20″S 151°54′50.40″E) in October 2011. Prior to commencement of experiments, P. sulcatus were collected from the field and kept in an aquarium with running seawater and were not fed throughout the duration of captivity. Only active (moving) adult P. sulcatus, with an average shell length of 2.5 cm were used for the experiments. All experiments were performed under controlled laboratory conditions in standard plastic food containers measuring 17 cm (L) X 10 cm (W) X 7 cm (H) containing still, fresh seawater filled to a height of approximately 3 cm (H). Two treatments: addition of crushed P. sulcatus juice in seawater (treatment) or addition of fresh seawater (control), were each replicated six times and assigned at random to individual P. sulcatus specimens. Each specimen was used only once, subjected to only one of either treatment. P. sulcatus juice was prepared by crushing four live P. sulcatus with the shells removed, using a mortar in 8 ml of seawater. Individual P. sulcatus were placed in the middle of the treatment container and allowed to rest (i.e. foots stuck to the bottom of the container and antennae extended) before any treatment were administered. Before each experiment, the containers were washed and filled with fresh seawater to remove any remnant odour from the previous experiment. 1 ml of juice or seawater were introduce into the experimental setup from the bottom right corner of the container, with a single individual being tested each time. The behavior of each test individuals was observed and any movements made were tracked using a marker, timed and recorded. An individual was deemed to have completed executing a behavior when it remained at a position motionless for > 3 minutes. In control treatments, where individuals were just moving randomly, without any clear direction or motive, the movements of the individuals were tracked over a period of 15 minutes. A picture is then taken of the path traveled by the individual and the distance covered by the individual is calculated using ImageJ image processing program as shown in Figure 1. The speed of the individual is obtained by dividing the distance travel over the time taken to cover that distance. Data analysis was performed using Student’s T-test to determine any statistical significance.
|
|
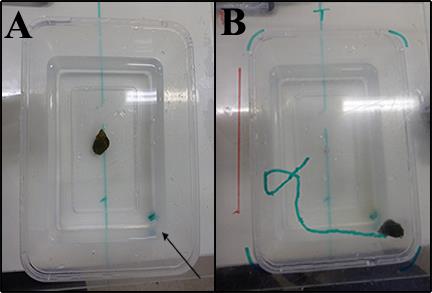 |
|
|
Figure 1: Experimental Setup. (A) Starting position: Test animals
were allowed to rest while being placed in the middle of the
container before any treatment were administered. The arrow
indicates the site (right corner) at which juice / sea water was
being introduced into the experimental system. (B) Movements
made by the test animals were tracked using a marker, timed and
recorded. Distance covered by the individual is calculated using
ImageJ image processing program.
|
|
Observed responses of P. sulcatus to crushed conspecifics
With the addition of crushed P. sulcatus juice, all (n=6) of the test conspecifics were observed at first to be highly attracted to the point source in which the juice was introduce, before moving rapidly out of the water until they were no longer submerged (Table 1). This observation was not observed in the control groups, where test P. sulcatus were either not responding (i.e. not moving) (n=4) or moving in all directions at random (n=2) (Table 1). Average movement speed of test P. sulcatus individual in the juice treatment group of 3.19 cm min-1 upon introduction of treatment was significantly faster (p< 0.001) than test individuals in the control treatment groups where movement speed averaged only at 0.15 cm min-1 (Figure 2).
|
Table 1: Response of P. sulcatus to crushed conspecifics. All (n=6) test P. sulcatus were attracted to the source of juice before moving out of the water until they were no longer submerged. P. sulcatus in the control group were either not responding (n=4) or moving at random (n=2).
|
Response
|
Attraction
|
Rejection
|
Random
|
No Response
|
No remain submerge in water
|
Treatment
|
6/6
|
0/6
|
0/6
|
0/6
|
0/6
|
Seawater
|
0/6
|
0/6
|
2/6
|
4/6
|
6/6
|
|
|
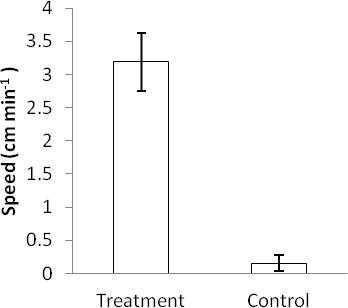
|
|
|
Figure 2: Movement speed of P. sulcatus in response to crush
conspecifics . Average movement speeds of P. sulcatus in
response to effluent of crush conspecifics is significantly
faster than individuals in the control group (p<0.001) .
Data were presented as mean + SEM.
|
|
Discussion of observed response by P. sulcatus to crushed conspecifics
Observations from this study clearly demonstrate that the response of P. sulcatus in Heron Island do respond to damaged conspecifics and they respond in similar fashion to that observed in Fiji but unlike those observed in Hong Kong (Lam 2002; McKillup & McKillup 1993). In response to effluents of conspecifics, P sulcatus from this study were observed to be highly attracted to the effluent in the beginning, before moving rapidly out of the water until they were no longer submerged. As the container used for this study had no crevices that the snails could seek shelter in, the observation whereby the snails moved rapidly until they were no longer submerged, was similar to the way which populations of P. sulcatus in Fiji responded in the event that the snails could not find or reach a crevice in the rocks to hide (McKillup & McKillup 1993). When P. sulcatus were found in their natural rock pool habitats, it was reported that in response to species-species signaling substances present in the effluent from damaged conspecifics, P. sulcatus would move rapidly in all directions, only stopping if they reached a crevice while still submerged and moved into it (McKillup & McKillup 1993). This strategy adapted by P. sulcatus in responses to crushed conspecifics has been suggested to be advantages in avoiding predation by shell-crushing predators as snails occupying a crevice cannot be easily dislodge and crush as on a smooth surface (McKillup & McKillup 1993). Moving upwards and out of the water until no longer submerged, would be the next most probable strategy to escape predation P. sulcatus could adapt, especially if the predator is never found out of the water (McKillup & McKillup 1993). The increased in movement speeds was also evidence to suggest that P. sulcatus on Heron Island does respond to damaged conspecifics as increase movement rates is also one of many response that is part of prey escape and avoidance response (Lam 2002; McKillup & McKillup 1993).
It is understood why in this study, P. sulcatus were attracted to the effluent from damaged conspecifics before adapting an escape response instead of adapting the avoidance/escape response immediately upon detection of alarm signaling substances in the effluent from damaged conspecifics as observed in previous studies (McKillup & McKillup 1993). A probable explanation would be that the effluent had remnants of food present in the guts of the crushed conspecifics and being not fed prior to the start of the experiment, test P. sulcatus may be attracted to the food before realising that there may be the impending danger of being predated. As it subsequently detects the inter-species alarm signaling substance present in the area, it switches to adapting an escape response in order to avoid being predated potentially .
Prey avoidance and escape responses of invertebrates are not simply generalised reactions to contact with predators but instead are specialised and effective responses which a species had evolve to cope with its predators effectively and especially when encounter rate between predator and prey is very high (Jacobsen & Stabell 1999; Lam 2002; McKillup & McKillup 1993) . For populations of P. sulcatus in Fiji and Heron Island to developed such similar and specialised prey avoidance and escape response to effluents from damaged conspecifics but not developed in population of P. sulcatus in Hong Kong, it is logical to imply that populations of P. sulcatus in Fiji and Heron island are facing far greater selective pressures imposed by increased predation pressure in these area as opposed to the population in Hong Kong and thus reflects indirectly, the amount of biodiversity in these regions .
Due to the short duration spent on Heron Island on this field trip, it was not possible to investigate if escape responses in the Heron Island population of P. sulcatus are similar to that observed in the Fiji population when being exposed to their natural beachrock/ rock pool habitat in the field. Future studies could be done to investigate the behavior of Planaxis sulcatus in response to affluent of conspecifics under natural field conditions.
|
|
|